Dr. Shirley Meng: The Push for Petajoule-Scale
Dr. Y. Shirley Meng, is one of the world’s leading experts in nanomaterials for energy storage. She is a professor at the Pritzker School of Molecular Engineering at the University of Chicago and Chief Scientist for Argonne Collaborative Center for Energy Storage Science.
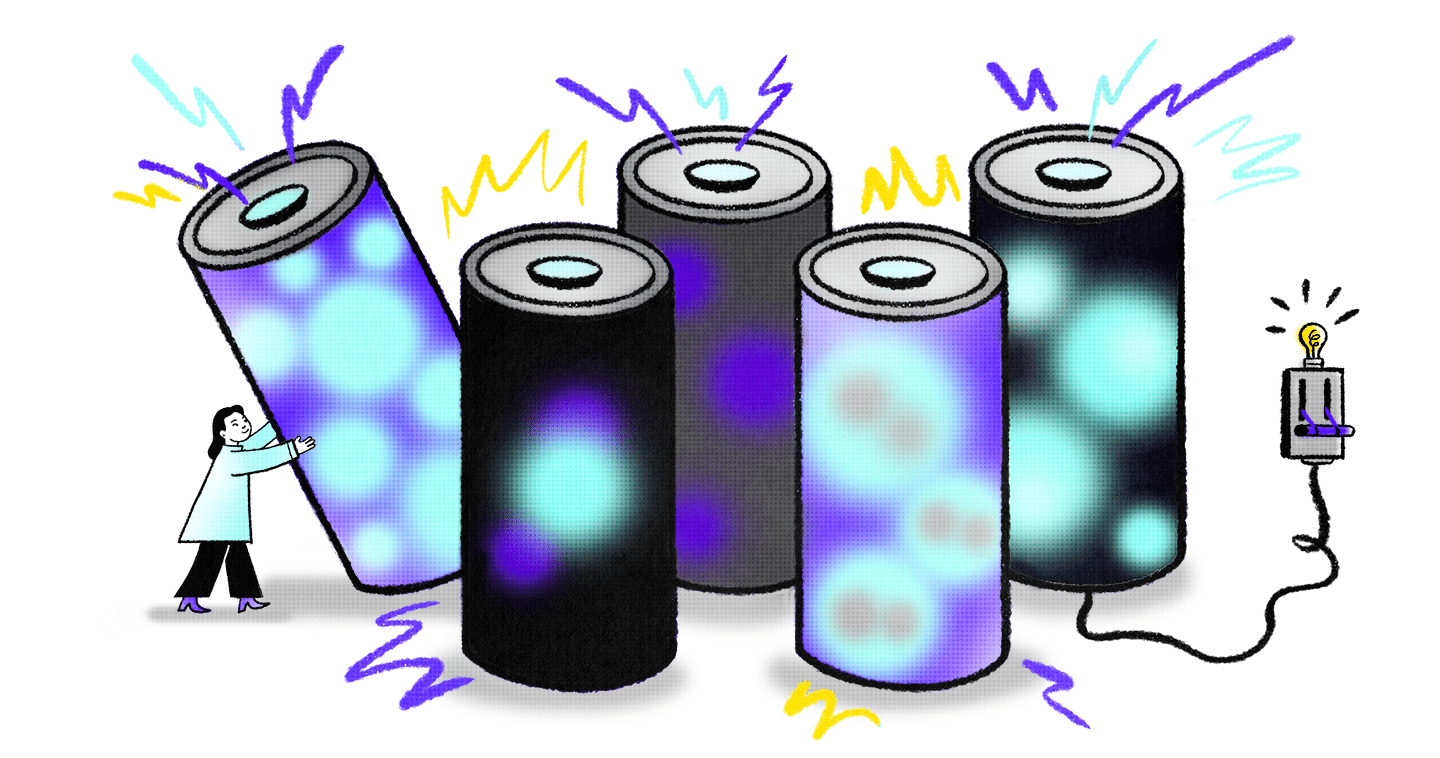
What does the future of energy materials look like from your perspective?
I think the future lies in how we make battery compounds. How can we sustainably make those battery-active materials—nickel, cobalt, lithium, sodium—and then be able to recycle them? So, kind of closes the loop as a circularity. Once you take it out from the mines, they will circulate in the world in perpetuity. That implementation has proven to be extremely difficult because of the way materials are extracted from mines and how they are produced and transported.
Looking into the future, I think we won’t have a bright future if major industry does not come on board to this energy transition. That must happen because, in my mind, I don’t doubt the transition will be solar and wind together with hydro, battery, thermal storage, and maybe a bit of nuclear. But the picture is that the whole infrastructure must change. The mindset has to be that batteries are infrastructure, not disposable.
How urgent is it to develop new compounds and new types of batteries? What will it take to electrify the world?
Our estimate is that we will need 200 to 300 terawatt-hours of battery capacity to electrify the world. Right now, I think the world is producing about two terawatt-hours of batteries per year. One terawatt-hour is merely enough to power the United States for one hour. The current estimate does not even include industrial needs from the grid, but just for mobility, and other small things. So, I think everybody should be asking themselves how many batteries we will need to have a world that is electrified and how to scale this faster.
Lithium-ion batteries have become the dominant technology for electric vehicles and portable devices. Why are these batteries so dominant and do we need alternatives?
It’s functionality and absolutely the properties of lithium. Lithium is light and reactive. It doesn’t take much space so it can give high-energy density in terms of volume and weight. The lithium-ion battery was the first single cell battery that reached four volts and could turn on all the transistors at once and be rechargeable. That’s what the wireless world and mobile world need. That’s what revolutionized everything.
But when we look at the lithium, we do get worried because we have much, much less abundant lithium and then also many industries compete for lithium. If we want to build so many gigawatt factories, we’re going to run out of cheap lithium, we’ll run out of nickel, and out of copper. So, we always question ourselves because we need to reach petajoule-scale: Do we have enough of these materials?
Part of this is about finding alternative materials and the other part is about building better batteries. How far are we in exploring alternative materials for future batteries—where are our successes and what are the key challenges?
I think the materials discoveries of the last few decades, the most exciting thing is the ability to control matters at a nano and molecular level. We know things behave differently in the nanoscale compared to the bulk and we can actually access the unique capability of materials when we control them and place them in the right position at molecular level. So, I think the future direction is looking at bringing those capabilities to scale.
To me, the biggest challenge is that how do you use these properties billions of times, with the same accuracy and the same precision. In energy storage, we haven’t really broken the cycle of new discovery to implementation. If we do find a new combination of elements, new arrangement of atoms and molecules, or create new properties, how can we accelerate that to impact at the device level much earlier? That’s a major challenge for all of us, because typically, it takes more than 15 years, but we don’t have that time.
“I think everybody should be asking how many batteries we will need to have a world that is electrified.”
There are many ideas of what the next generation of batteries might look like—lithium-air, lithium-sulfur, sodium-ion, and flow batteries. From a materials perspective, which of these seems the most promising, and why?
If you ask me which technology will succeed, in my opinion, all of them have to succeed, every single one of them.
Hopefully, the sodium batteries will be the first ones to reach terawatt-hour within 10 years. The next one, I believe lithium metal batteries will be successful, and they will be used in niche places first like flying drones and possibly some very high-end power tools. Perhaps that will give us enough time to make lithium-sulfur and metal oxygen batteries succeed before the mid-century. I think all solid-state batteries probably will rise up very quickly in the next decade after 2030.
The world will need hundreds of terawatt hours from batteries. We’re not saying other technologies, like hydrogen, won’t help. But that’s what the battery world should be striving for. If we just rely on lead-acid and lithium-ion, we will never get there. So at least two or three more battery technologies have to come and succeed.
What are the key properties that will make future batteries and materials?
If you are asking me about the future, I think the future relies on batteries that go completely solid—solid electrolyte instead of the liquid or polymer gel that are currently used in lithium ion batteries. All that liquid is the problem we have when we scale, and a lot of the safety of the batteries is caused due to the flammable liquid electrolytes.
The roadmap is quite clear, but I am not sure of the roadmap of actual materials. The semiconductor industry could be sustained so well because we have silicon, right? So when we look for materials, the number one thing is the geology tables. Do we have enough and can I scale to terawatt hour, to petajoule? If my battery’s main element is sodium, I don’t think anybody is going to worry about its abundance. So combining sodium and solid-state is a goal.
We also want to build batteries for grid storage directly that are capable for 100% of deep discharge, capable of being very safe, never catching fire, and 100% recyclable. We need to have these batteries as a part of the infrastructure that lasts for 30 years, just like solar panels give a 25-year guarantee. So that’s the kind of batteries we’re working on—not a decade-long battery but building batteries that last for three to four decades.
EV battery recycling and second-life uses are hot topics in the battery world. What are the challenges with recovering, reusing, or reconfiguring existing batteries in a circular economy?
It all comes down to if you can accurately assess if the battery is still in good condition and predict how much more lifetime the battery can have safely. You must ensure that before it fails catastrophically, it will be end of life.
Right now, batteries are all just brutally recycled. And I don’t think that’s the best use of the end-of-life batteries. We still have a lot of available energy inside the batteries. So going directly for recycling, it’s probably not the smartest thing to do. In the next three years, we will have to make a decision on what to do with a lot of retired batteries.
Perhaps there will be methods coming out that we can regenerate batteries through certain electrochemical or chemical means. This is an active research area a lot of scientists are working on, similar to self-healing materials. Maybe in the future, you will be able to send your battery back for service, just like a car. Right now, it’s still like science fiction, right? But there are active programs now that are trying to make that happen.
If you had to pick the top problems for materials scientists to work on, other than batteries, what would they be?
I would say that green hydrogen is already being produced very cost-effectively. But with hydrogen, the main thing is really how to store and transport it. Ammonia is an old technology. I don’t think anybody wants to live in the neighborhood where a huge amount of ammonia is stored. So production is not the problem, and I hope materials scientists will ride this wave to do good things in hydrogen transport and storage.
The second game changer is CO2 capture. The problem is not the capture. The problem is what do you do with the captured carbon. I think a materials scientist better figure this out because I just don’t see how direct air capture or point capture of CO2 doesn’t eventually get released back to the air. If I was starting as a materials scientist today, that’s the area I would focus on—what can you make with CO2? That’s the grand challenge.